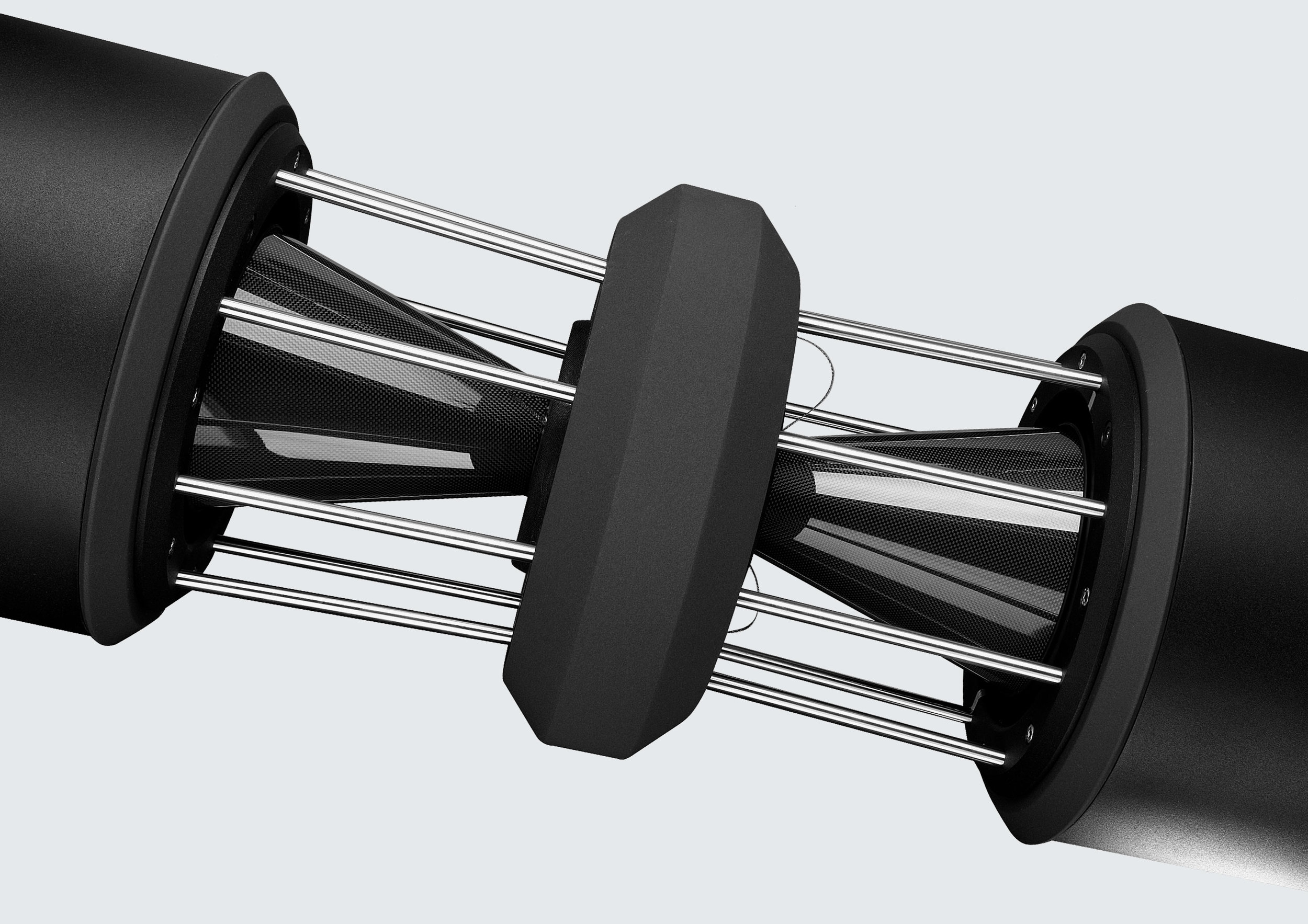
At first glance the German Physiks DDD driver looks like a conventional pistonic cone driver. It has a voice coil/magnet assembly that serves as the actuator and it has a cone, though this is longer and narrower than usual. The shape is where the similarity with a pistonic driver ends
With a pistonic driver, when the voice coil moves, the entire cone moves together with it – or that is what we want it to do. This is why the cone and voice coil structure is made as rigid as possible. The sound wave that a pistonic driver produces moves in the same direction as the movement of the cone - figure 1. This is why pistonic drivers are generally placed facing towards the listener.
Figure 1
The DDD driver, despite its apparently simple appearance is rather more complex. It has 4 regions of operation and in essence works as a mechanical 4-way system
1. The lower frequency end of its operating range can be described with Small/Thiele resonant parameters.
2. In the next frequency band up to the Coincidence Frequency, it works like a pistonic driver.
3. Next, an overlapping band follows where pistonic movement is progressively replaced by bending waves until all the radiation is generated purely by bending movement in the cone. Due to dispersion and the cone’s special shape, the Coincidence Frequency is spread over an extended frequency range, rather than occurring at a single frequency like the Dipole Frequency.
From the upper edge of the Coincidence Frequency band, it works like a pure bending wave converter where the velocity of the travelling waves in the cone increases with frequency.
4. The last mode of operation commences above the bending wave band at the Dipole Frequency, when the first standing wave occurs and where modal break-up begins.
By optimising the key properties of the cone material, namely thickness, elasticity and specific weight, together with the cone’s bending stiffness, all four frequency bands may be very closely balanced.
The last two regions cover the majority of the DDD driver’s operating range and are what differentiate it from conventional drivers. In these two regions, when the voice coil moves, the whole cone does not move together with it, as the open end of the cone is terminated by a rubber suspension and semi-rigidly attached to the driver chassis. Instead the motion of the voice coil causes a wave to travel from the top of the cone down to the open end. This occurs because unlike the pistonic driver, the DDD driver cone is made from a very light and flexible foil – 0.15 mm thick carbon fibre. While the shape of the cone gives it rigidity at rest, it is relatively easy to excite waves in the cone material. The clever part is controlling these waves. In very simple terms, the resulting motion can be compared to that of the bell of a jelly fish when it is swimming, with the sound wave radiated sideways from the driver as shown in figure 2. For this reason the DDD driver is always mounted vertically. The movement of the cone is rather more complex, as the angle of radiation with respect to the cone wall becomes progressively more acute with increasing frequency due to dispersion of the bending waves and in addition, the driver’s operating mode changes with frequency.
This type of driver is sometimes referred to as a transmission line driver because the cone carries a wave and so acts as a transmission line. We prefer to call it a bending wave radiator. Bending waves describe the type of wave set up in the cone by the actuator motion. The use of this nomenclature also avoids confusion with the acoustic path transmission line loudspeakers that most audiophiles will be familiar with, such as in the classic designs from Paul Voigt, A. R. Bailey and TDL®.
Optimising the DDD Driver
The DDD driver utilises a powerful ferrite magnet of our own design together with an under hung voice coil. The magnetic strength in the gap is approximately 1.2 Tesla. This gives the DDD driver a sensitivity that is on a par with that of conventional cone drivers of similar dimensions. The very high magnetic strength in the gap also provides a useful increase in efficiency in the upper two operating bands.
The linearity of the DDD driver’s magnetic circuit – a parameter that is crucial to the performance of any dynamic driver – is also extremely high and we believe it to be one of the best in the industry. This ensures that the force that the voice coil produces to drive the cone very accurately follows the input audio signal.
To limit the moving mass, the weight of the voice coil must be minimised, consequently the upper frequency limit and maximum power handling become issues. In early prototypes, the upper frequency limitations posed quite a challenge.
Today we achieve a power handling capacity comparable with that of conventional woofers by using a voice coil constructed with flat wire that is wound on its edge, allowing an extremely densely packed winding and also by completely enclosing the coil within the magnetic structure, which then serves as a more efficient heat sink for the voice coil.
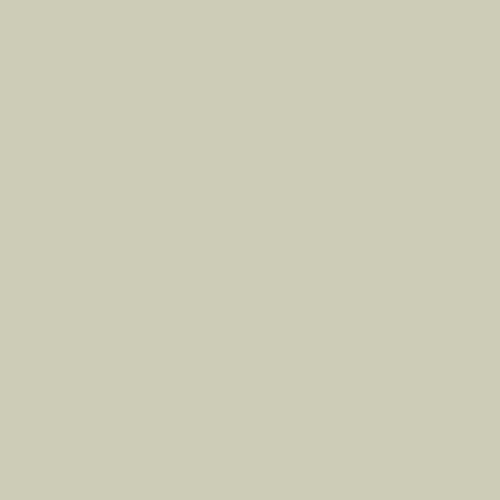
Controlling the Cone
In any driver of this type you want the motion of the cone to precisely follow the electrical signal that drives it
You do not want the cone to ring. This is where the cone continues to move after the impulse that excited it has finished. Essentially perfect control of the cone and an absence of ringing are easily achieved when the wavelength of the frequency propagated down the cone is greater than the length of the cone itself. It is difficult when the wavelength is shorter, since the full wave is reflected from the boundary of the cone – this is where the end of the cone opposite the voice coil is mounted to the driver chassis. The reflection will in turn produce re-reflections as the travelling wave slowly loses energy over the course of several wave cycles. Imagine small ripples in a pool and how they are reflected back from its edge in a recurring pattern. The behaviour of a rippling cone is precisely the same, as the motion tends to persist for a considerable time, which ultimately has the effect of obscuring the information being reproduced.
Our own approach to this problem is two pronged. Firstly, Peter Dicks found that bending waves disperse, i.e. the DDD driver cone exhibits an increasing velocity of propagation of waves (more correctly termed phase velocity) with increasing frequency and he was able calculate the velocity of propagation in the cone as a function of frequency and therefore determine at what frequency the velocity of a wave in the cone reached that of sound in air. This is called the Coincidence Frequency. At this frequency the waves start to detach from the cone’s surface at an angle given by the ratio of cone-speed to air-speed. A few Hertz above the Coincidence Frequency the detachment angle is close to zero, but with higher frequencies the elevation approximates to 90°. The detachment of waves from the cone describes the functional principle of the bending wave radiator. Secondly he devised an effective means of balancing damping of the cone and the characteristics of the cone termination in order to minimise the residual ringing in the cone.
The calculations required to determine this were extremely involved, which is why to the best of our knowledge, no one prior to Peter Dicks had been able to devise and solve the necessary equations
Whilst the DDD driver’s cone looks like a simple enough shape, this is deceptive because the behaviour of waves in the cone is anything but simple. Not only does the cone exhibit dispersion (different frequencies travel at different speeds), but the stiffness of the cone is not constant along its length. In fact it decreases as you move from the peak to the mouth (open end). Consequently the velocity of a wave in the cone is proportional to frequency and inversely proportional to the distance from the cone’s peak.
The carbon fibre material we use for the cone material now and the titanium foil we used in the past both have a high velocity of propagation in their bulk form. The velocity of propagation is especially high when they are formed into a very steep, thin walled cone. As mentioned above, the velocity of propagation in a cone varies along its length, being highest at the peak and slowest at its mouth.
This means that the upper part of the cone reaches the Coincidence Frequency much earlier than the lower part, and additionally, in the lower part the wave lengths are shorter and all the waves are denser and therefore more efficiently radiated into the surrounding air. This higher efficiency of radiation means that there is less energy left in the cone to be reflected at the termination (i.e. the surround) and by maximising the amount of energy being radiated a more simple form of termination for the cone may be used. This is important as the reflections would otherwise cause ringing in the cone. The theoretical way to eliminate this would be to ensure that all the remaining energy in the wave was absorbed by the termination, but this can only be done by having a termination that is the complex conjugate of the cone’s impedance and this cannot be physically realised.
Another advantage of the DDD driver is that because it produces bending waves in a wide frequency range above the bass region, movement of the cone caused by bass signals produce almost no Doppler distortion, the bane of conventional loudspeakers
The material we use for our carbon fibre cones is 0.15 mm thick and the material we used to use for our titanium cones was 0.025 mm thick. These materials in their sheet form are very delicate and must be handled with great care. Once formed into a cone they become more manageable. Nevertheless, assembling a DDD driver is a slow and pains taking process that can only be performed by skilled technicians. We have experimented with even thinner materials, but have found the resulting structures extremely fragile and have concluded that the present dimensions give the best compromise between efficiency and performance on the one hand and strength and maximum power handling on the other.
With its current dimensions, the DDD driver reaches the Dipole Frequency below 4kHz for the carbon fibre cone version and around 6kHz for the titanium cone version. At this point the first standing wave starts to build up and above this frequency, the wavelengths become progressively shorter than the cone.
Once beyond the Dipole Frequency, dispersion and radiation work as damping mechanisms to control the motion of the cone, however, additional damping may be employed to smooth the pulse and frequency response even more. These control ringing very effectively and allow the carbon fibre DDD driver to operate to over 24kHz.
The Free Lunch
The DDD driver mounted vertically possesses an added advantage that is arguably just as great as the unrivalled linearity of the design itself. That is, it is a nearly ideal point source with an omnidirectional radiation pattern.
The DDD driver propagates sound in a uniform spherical pattern. The frequency and phase responses are uniform from all listening angles, which is never the case with multi-way cone and dome loudspeakers, nor with dipole electrostatics or ribbons.
An omnidirectional radiator has several audible advantages:
1. The window in which stereo imaging will be perceived is considerably widened, and “head in a vice” listening constraints are much relaxed.
2. The loudspeaker’s behaviour tends to be much more predictable from room to room, because the reflected sound is timbrally matched to that of the direct sound.
3. The sound of an omnidirectional loudspeaker has decay characteristics more closely resembling large room reverberation than is the case with the narrowly focused output of typical monopole direct radiators. The sound has a naturalness about it that powerfully suggests the experience of a live musical performance.
It is possible to approach such a radiation pattern with arrays of conventional drivers and certain types of diffusers and horns, but only the DDD driver achieves perfect omnidirectionality at all frequencies and at no penalty. Yet another unique attribute of the world’s most nearly perfect loudspeaker.
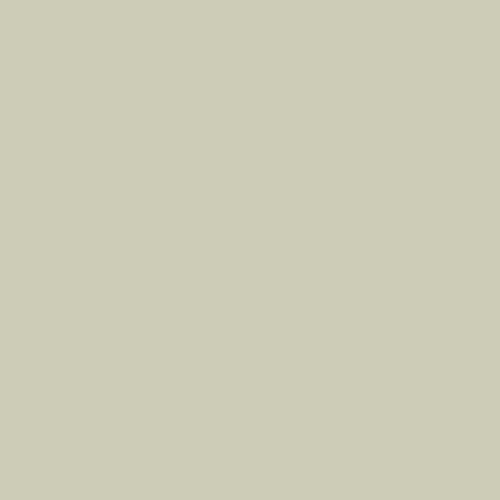
Why is the DDD Driver Truly Revolutionary
To answer this question we first need to look at the principle types of loudspeaker driver currently available. All loudspeaker drivers may be categorised by two groups of fundamental characteristics.
Group 1: This describes the means of electromechanical transduction; that is the method by which electrical energy representing the audio waveform is converted into mechanical energy and this in turn breaks down into three basic types
Electromagnetic or electrodynamic
A simple reciprocating motor drives the cone, diaphragm or sound producing element. Well over 95% of loudspeakers sold today fall into this class, which includes the DDD driver; its one point in common with conventional designs.
Electrostatic
Air motion is produced by varying the electrical charge on a diaphragm located between two electrodes.
Piezoelectric
This employs materials which flex in one dimension when an electric potential is applied across them.
While other methods of transduction such as magnetostriction and corona discharge modulation have on occasion been employed, none has achieved commercial significance, so the three categories above cover almost every loudspeaker currently available.
Both the electromagnetic and the electrostatic types can offer high levels of linearity. The piezoelectric type has generally been confined to applications where high sound quality is not a requirement.
Electrostatic loudspeakers are held in high esteem by many audiophiles, and deservedly so on the basis of their sound quality at moderate output levels, but they suffer from a number of serious limitations:
Restricted maximum output level especially at low frequencies
Medium to low electrical efficiency
High directivity particularly at high frequencies
Poor reliability especially in humid environments
They can be difficult loads for some amplifiers.
We have done extensive research on electrostatic loudspeakers, but have concluded that they are inherently impractical and unlikely to progress much beyond their current state of development. In consequence of the above, the electromagnetic type is in our view the transducer of choice.
Group 2: This fundamental group of characteristics deals with how the mechanical energy is converted into acoustic energy and breaks down into the following types
Mass loaded pistonic drivers.
Tympanic or membrane drivers.
Flat panel loudspeakers such as the NXT® and BMR® devices.
The Heil® air motion transformer.
Transmission line drivers, chiefly represented by the Jordan Module® and its developments; the Manger® driver; the Walsh® driver and the German Physiks DDD driver.
Mass Loaded Pistonic Drivers
This includes nearly all conventional cone and dome drivers
The name itself refers to the dominant effect of mass on the acoustic output of the driver, and to the theoretical model of pistonic motion to which such drivers conform to a greater or lesser degree. According to this model, the driver actuator and diaphragm (usually a cone or dome), should move back and forth as a single unit within a single dimension, just like the piston in a reciprocating internal combustion engine. Ideally, the diaphragm of the driver should remain entirely rigid and should exhibit no internal vibration whatsoever, though in practice this condition is never met, except at the lowest frequencies.
In such designs, mass reactance will be the main component of the complex acoustic impedance of the diaphragm throughout most of the useful frequency range of the driver, hence the term, mass loaded. The mass in turn is loaded by the compliance or springiness of the driver’s suspension, and the two together, mass and compliance, form a resonant system like a weight suspended from a spring. Such a system tends to oscillate around a single frequency when excited, and, predictably, a large part of conventional loudspeaker design is taken up with damping such oscillations.
Unfortunately, a resonant system, even a damped resonant system, is poorly suited to sound reproduction.
Audible sound spans 10 octaves, while a strongly resonant system is mechanically efficient over only a small range of frequencies close to its resonant frequency. Such systems are necessarily bandwidth limited due to this frequency dependent efficiency, while transient response is inevitably degraded due to the inertia of the mass and because of the energy stored in the loudspeaker’s suspension and which is returned subsequently.
Such a driver, unless it is loaded into a horn or acoustic lens, will also suffer from a non-constant directivity pattern, with dispersion normally narrowing with increasing frequency. This is often called beaming. This characteristic, perhaps more than any other, will cause a loudspeaker to sound musically unnatural since acoustic musical instruments almost never radiate sound in this manner.
The normal method for dealing with the limitations of mass loaded pistons is to use two or three per speaker system and to drive them over the narrow frequency ranges around their respective resonant frequencies. Many fine speaker systems have been designed this way, but we believe this approach is fundamentally flawed, and nearly always results in location dependant transient response, ragged directivity patterns and an overall sense of individual drivers imperfectly integrated.
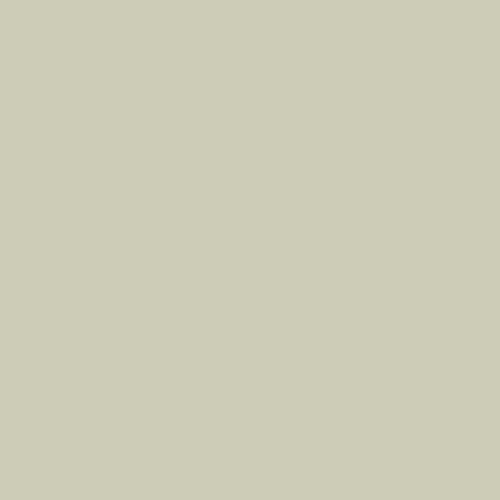
Tympanic or Membrane Drivers
This includes film and leaf transducers, electrostatic and ribbon type dynamic loudspeakers
All of these use a diaphragm that has a low mass and a large surface area and which is stretched over a frame; in other words the diaphragm and suspension are one and the same. In such designs the resistance of air dominates the acoustical impedance of the diaphragm except at the lowest frequencies. Consequently transient response and frequency range can be excellent. These designs normally lack high power handling capabilities and are incapable of large excursions, so they are best confined to the higher frequency ranges and indeed are thoroughly impractical in bass applications. Moreover, they typically dictate a line source configuration which makes them difficult to use in domestic listening environments. They also tend to be difficult to integrate with mass loaded pistons due to their very different acoustical characteristics. As a result hybrid systems incorporating them very rarely provide satisfying results.
Flat Panel Loudspeakers
The NXT® driver can be described as a special membrane driver where its technology is based on the modal excitement of a stiff panel diaphragm where a number of areas are stimulated, but using a single exciter
The number, location and size of these areas vary with frequency. While optimising the location of the exciter point for a given panel may flatten the frequency response of the loudspeaker, it always lacks the phase coherence and wide dispersion of energy which are mandatory for high fidelity loudspeakers.
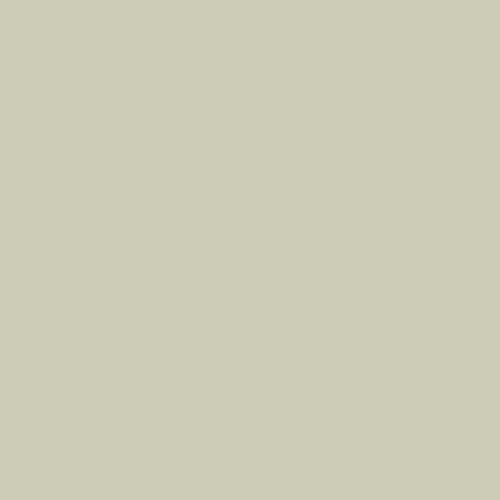
Balanced Modal Radiator
This technology, a spin off from NXT®, is based on pistonic movement, where the area of radiation becomes progressively smaller with increasing frequency
It uses a single exciter, but in contrast to the NXT® panel, only one vibrating area is employed and the size of this area varies inversely with the frequency of the stimulus.
The Heil Air Motion Transformer
This occupies a class of its own and is worthy an essay in itself
It is an extremely ingenious design with high output, high efficiency, wide bandwidth, good impulse response, and low distortion. Its sole real drawback is its low frequency limit - not much under 1kHz - and the consequent necessity of mating it with a conventional woofer. Sadly, most attempts at doing so have resulted in audible mismatches.
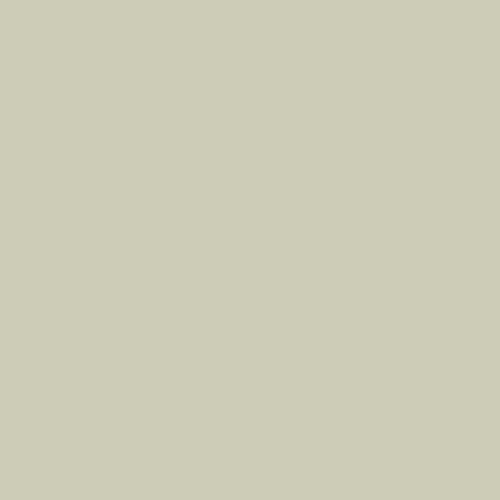
The Transmission Line Driver
This is the class that our DDD driver falls into, which when optimised offers what is currently the best overall performance in sound reproduction
Whilst similar in appearance to a mass loaded pistonic driver because of its conical diaphragm and use of a conventional voice coil and magnet type actuator, it differs in one major aspect. The cone is securely anchored at its mouth and is flexed by the motions of the voice coil, and only pushed back and forth up to its Coincidence Frequency. From there on sound propagation is at angles up to 90 degrees to the wall of the cone, rather than parallel to the path of the voice coil, as is the case in the lower pistonic frequency range.
The cone itself ideally has an extremely high stiffness to mass ratio, but because it is very thin and its moving mass is extraordinarily low, so also is the bending resistance. Consequently, the cone will be excited into bending modes quite easily, particularly when the velocity of the waves on the diaphragm is higher than the velocity of sound in the surrounding air and consequently the wave energy will detach from the cone surface.
Without going into the physics of traverse wave propagation across a plate structure - which essentially is what the cone is in this design - we can say that when the cone is bent by an actuator, the actuator itself - in this case the voice coil - sees only a very small increment of mass from the cone.
Rather than being mass loaded, it is loaded instead by the differential stiffness per specific weight of the cone-material and secondarily by the radiation resistance of the air load on the cone.
In simple terms, the voice coil is exciting shock waves across the surface of the cone which in turn excites motion in the air. As distinct from a conventional cone, there is almost no mechanical inertia to overcome, thus there is a very direct translation of the electron motion of the audio signal into the motion of air molecules in the listening space.
In a real sense, the acoustic behaviour of the system is much closer to that of an electrostatic membrane speaker than to a mass loaded cone, to which the transmission line driver bears a misleading external resemblance. The moving mass of the German Physiks DDD driver is under three grams, less than that of most tweeters, and yet its ability to displace air is roughly equivalent to that of a 6.5-inch woofer. So while it shares the cone shaped diaphragm of the latter, its behaviour could not be more different.
Conclusion
To come back to the original question, why is the DDD driver so revolutionary?
At a stroke most of the limitations of conventional drivers have been eliminated. The combination of high displacement, low mass, and high acceleration allows the DDD driver to operate linearly over a very wide frequency range, nearly the whole of the audible spectrum and to achieve excellent impulse response, low distortion and a flat phase response into the bargain. The German Physiks DDD driver is able to offer an improvement in sound reproduction that we feel quite justified in describing as revolutionary.
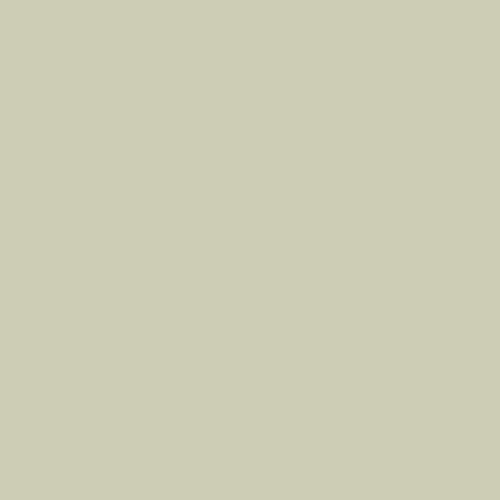